Pressure ulcers (PU) are generally defined as any lesion involving the skin, subcutaneous tissue, muscle and/or bone. A PU usually occurs over a bony prominence in long-term, bed-bound patients. A PU can develop within 24 hours as a result of pressure alone or pressure combined with shear and/or friction, and may take as long as five days to appear.1
The intensive care unit (ICU) is generally the hospital department to care for patients who have failure of one or more vital organs or haemodynamic instability. Such patients require specialist intensive medical care and continuous nursing and monitoring. Thus, the ICU is a environment favourable to the emergence of PUs.2,3 The published rate of PUs in ICU patients ranges from <1% to >50%, with an estimated 63% mortality rate for patients with PUs in the ICU.4,5–6
PUs can negatively impact on a patient's quality of life (QoL) while also increasing the risk of nosocomial infections.7 Impaired recovery may contribute to a higher mortality risk in patients and greatly increase nursing time and treatments costs.7
Once a PU has developed, immediate treatment is required. Commonly used treatments have included debridement, wound cleaning, ointments, creams, solutions, antibiotic therapy, nutritional support, dressings, ultrasonography, ultraviolet heat lamps and coverage with compressive or non-compressive bandages.8 When choosing a treatment strategy, consideration should be given to the stage of the wound and the purpose of the treatment (for example, protection, moisture or removal of necrotic tissue).8 Category I and II PUs usually heal within several weeks with conservative and appropriate general care of the wound, whereas Category III and IV PUs are more difficult to heal. Hyperbaric oxygen therapy, ketanserin, cultured human dermis, electrical stimulation, platelet (PLT) releases and growth factors (GFs) are new-generation treatment methods that can provide healing acceleration, owing to their proliferation-eliciting activities, especially for treating foot ulcers in patients with diabetes, chronic ulcers and wound healing.9,10,11,12,13,14,15,16–17
Topical dressing with PLT releases is a wound healing treatment that has been successfully used since 1986.18 Randomised controlled trials have also demonstrated recombinant human growth factors to be effective in treating difficult-to-heal wounds, such as chronic neuropathic ulcers and foot infection in patients with diabetes.19,20 A new blood component, PLT gel (made from differential centrifugation of whole blood, containing a large amount of autologous thrombin), was added to the therapeutic repertoire as an adjuvant to accelerate tissue regeneration in a variety of clinical settings. These include facial plastic surgery, bone regeneration, bone grafts and surgical management of macular holes.21,22,23,24,25,26,27–28 Although several studies have reported promising results for the use of PLT releases as an enhancer for tissue healing, there remain some patients with no clinical improvement.27 It may be that, in most of the cases, the PLT releases were obtained from a single blood donation, whereas the blood of different individuals has significant variability in platelet concentration and growth factor levels, and different individuals have different responses to treatment.27
Autologous platelet-rich fibrin (PRF) is an option for reducing inter-individual variability. Although studies have reported that it can serve as an enhancer for tissue healing and regeneration, no data exists on the efficacy of PRF in routine clinical PU healing. In this study, an autologous platelet-rich fibrin-based bioactive membrane for treating PUs in an animal model was developed. Additionally, the biological activity of the PRF bioactive membrane was investigated by measuring cell proliferation, migration and cell healing assays. The results presented in this paper will provide an experimental basis for its future clinical applications.
Method
Rat skin pressure ulcer model
This study was approved by the Laboratory Animal Research Center and Use Committee of the Zhejiang Chinese Medical University. The study was performed in compliance with international guidelines on laboratory animal protection for the care and use of animal models.
Healthy adult male Sprague-Dawley (SD) rats (weight range: 230–280g) were used. The animals were caged in a room with the temperature controlled at 25±2°C and had unlimited access to food and water. After establishing the PU, the rats were divided randomly into two equalsized groups: the control group and the PRF bioactive membrane treatment group.
Rats were placed individually in a Lucite chamber and exposed to an anaesthetic (3–5% of isoflurane mixed with oxygen) until unconscious. The fur was shaved and the skin disinfected, following which an incision was made in the midline of the back. Blunt dissection was carried out to expose the deep fascia, and a metal plate (diameter 4cm, thickness 2mm) was implanted subcutaneously. An external magnet (magnetic flux density of 1200 Gauss) was used to exert intermittent pressure. The external magnet attracted the transplanted disk, exerting pressure on the skin and resulting in localised skin ischaemia. A cycle of external magnet removal after every two hours of inducing ischaemia, followed by a partial restoration of blood flow for 30 minutes was performed. Each rat underwent four successive cycles per day for five consecutive days. A total of 20 cycles of ischaemia-reperfusion and 40 hours' of ischaemia time were needed to create the PU. To prevent potential infection, antibiotics were given at the preoperative day and the consecutive three days after surgery.
Preparation of the autologous platelet-rich fibrin-based bioactive membranes
Rats were individually placed in a Lucite chamber and exposed to an anaesthetic (3–5% of isoflurane mixed with oxygen) until unconscious. After the fur on the collection site was shaved and the skin disinfected, a catheter was inserted into the femoral vein of one leg below the inguinal ligament. An empty syringe was attached to a stopcock connected to an arterial catheter and then 5ml of femoral vein whole blood was collected. After collection, the blood was expelled into a sterile 10ml glass centrifuge tube. Within five minutes of collection, the whole blood samples were spun at 3000rpm in a refrigerated centrifuge (4°C) for 12 minutes. Samples were removed from the glass centrifuge tube and then left to stand for 10 minutes until they were separated into three layers: a clear serum layer, a platelet-rich fibrin (PRF) layer and a red blood cells solidification layer. The PRF layer and the solidified red blood cells were removed and put into a small, medical-grade, low-density polyethylene sterile bag (Zhonghui Pharmaceutical Packaging Co. Ltd., China). The bag was sealed, flattened on a table, covered with a weight of 50g and gently squeezed so it would become a thin PRF bioactive membrane for use in the study.
Extraction of rat autologous platelet-rich fibrin soluble factors and solution preparation
The soluble factor extraction was derived from the same autologous PRF preparations used for the PRF bioactive membrane. To break up the PRF and release the soluble contents, PRF and 5ml of rat endothelial growth medium (R211-500, Sigma, US) were put into a 15ml sterile tube and exposed to three freeze-thawing cycles. The tube was then placed on ice and homogenised (PRO Scientific Inc., US) at maximum speed of 1000rpm) in an ice-bath. The homogenisation buffer was centrifuged at 15,000g for 20 minutes at 4°C to precipitate the broken platelets, and the supernatant (the PRF soluble factor extraction) was aliquoted and stored at –80°C for future study of cell biological activity. The total protein concentration of PRF soluble factor extraction was determined by the bicinchoninic acid (BCA) method.
Cell culture
Rat aortic endothelial cells (RAOECs, R304-05A, Sigma-Aldrich Co., US) were cultured with rat endothelial growth medium (REGM, R211-500, Sigma-Aldrich Co., US). Before seeding the cells, the T-75 flask or cell culture dishes were pretreated with attachment factor solution (123-100, Sigma-Aldrich Co., US), for 30 minutes at 37°C. The cells were maintained at 37°C in 5% CO2 cell incubator and passaged the cells at 80% confluency every three or four days after seeded into the T-75 flask or cell culture dishes.
Cell viability and proliferation in vitro assay
A CCK-8 assay was performed on RAOECs to evaluate cell viability and proliferation. RAOECs were plated in 96 well plates (pre-treated with attachment factor solution for 30 minutes at 37°C) at a density of 8×103 cells/well. After 24 hours, the culture media was replaced by REGM supplemented with different components, and the plates divided into groups according to the level of total protein from the PRF soluble factor extraction:
- 5µg
- 10µg
- 15µg
- REGM without supplements was used as the control.
At different times (days one, two, three, four and five), 20µl of CCK-8 solution was added to each well. After four hours of incubation, the absorbance was measured at a 570-nm wavelength by a multimode microplate reader (TECAN Infinite M200, Switzerland).
Wound healing in vitro assay
For the wound healing in vitro assays, RAOECs were seeded in 48 well plates (pre-treated with attachment factor solution for 30 minutes at 37°C) at a density of 3×104 cells/well in REGM supplemented with 10% fetal bovine serum (FBS). The plates were divided into four groups, according to the level of PRF soluble factor extraction protein supplements. After reaching confluence, the monolayers were scratched using 0.1–10µl pipette tips, washed with phosphate buffer solution (PBS) and covered with 500µl of REGM containing the protein supplements from the PRF soluble factor extraction:
- 5µg
- 10µg
- 15µg
- The basal REGM without supplements served as a control.
After 24 hours post-scratching, the medium was aspirated and the cells were rinsed with PBS. A wound healing image from samples in each group was taken using a phase-contrast Olympus IX71 microscope (Olympus, Japan).
Migration assay
The cell migration assays were done in 24-well Transwell plates (8mm pore size, catalog number 3422, Millipore, US), as described in a previous study.29 The RAOECs were seeded in the upper chamber of the Transwell system with 8×104 cells/well in 100µl of Opti-MEM I Reduced Serum Media (catalogue number 1985062, Gibco, US), and the lower chamber was filled with 600µl REGM, with or without the supplements from the PRF soluble factor extraction. The 96 plates were divided into groups according to the level of total protein from the PRF soluble factor extraction:
- 5µg
- 10µg
- 15µg
- Basal REGM without supplements served as control.
After 24 hours incubation, cells remaining on the top layers of the inserts were removed by cotton swab scrubbing and cells on the lower surface of the membrane were fixed and stained with haematoxylin-eosin (H&E) staining. The cell numbers in five random fields (x200 magnification) were counted for each group of plates and the average calculated. The random field of visions were on the lower surface of the membrane where the cells migrated and counted under the microscope
Rat pressure ulcer model evaluating the effectiveness of PRF bioactive membrane in vivo
Rats with PUs, as established above, were randomly divided into two equal-sized groups: the control group and PRF bioactive membrane-treated group. The control group was treated with normal care, such as a clean, sterile covering with non-adherent dressing. In the PRF bioactive membrane-treated group, after cleaning and sterilising the PU sites, the sterile polyethylene bag (containing the freshly made PRF bioactive membrane) was cut open on one side. The PRF bioactive membrane-exposed side was placed over the PU site and covered with a non-adherent dressing to prevent the PRF bioactive membrane from drying. The PRF bioactive membrane was made fresh at every treatment point and re-applied every three days. Digital photographs and measurements (length and width) were taken at days zero, three, seven and 14 to document changes in wound size during treatment follow-up. All measures were compared with the data measured immediately post-treatment (day zero), and all values were used to define a trend line for PU resorption. Three of the rats in each group were euthanised on days three and seven, and the wound area was harvested and prepared for histological analysis. The remaining rats were euthanised at day 14 and their wound areas harvested.
Histology and immunohistochemical assay
PU site samples from three rats in each group were harvested at days three and seven, and the remaining rat PU samples were collected on day 14 after treatment. Samples were fixed with 10% neutral formalin made with PBS for 24 hours and paraffin-embedded to obtain tissue sections. Sections were cut at 4µm thickness for H&E and immunohistochemistry (IHC) staining. For IHC staining, the sections were deparaffinised, rehydrated and then rinsed with PBS. Antigen retrieval was carried out in 0.01m citrate buffer (pH 7.4) for three minutes using a high pressure retrieval method. The sections were then incubated with 3% H2O2 for 10 minutes, followed by 10% normal goat serum for 15 minutes at room temperature to block endogenous peroxidase and non-specific antigens. Additionally, sections were incubated with 20µg/ml goat anti-rat VEGF polyclonal antibody (catalogue number AF564, R&D Systems Inc., US) overnight at 4°C. After rinsing with PBS three times, the signals were detected by using Histofine Simple Stain Rat MAX Peroxidase kit (for rat tissue and goat primary, catalogue number 414331F, B-Bridge International, Inc., US), according to the product's manual. Finally, the sections were counterstained with heamatoxylin.
Western blot (WB) assay
The RAOEC cells (treated with or without PRF soluble factor extraction) and the rats' skin PU tissues (PU site samples from three rats in each group were harvested at days three and seven, and the remaining rats' PU samples were collected on day 14) were collected and lysed in protein lysis buffer (1% NP-40, 20mM Tris-HCl (pH 8.0), 137mM NaCl, 10% glycerol, 2mM EDTA). Then, 40μg of total protein was used for WB, along with 0.1µg/ml of goat anti-rat VEGF polyclonal antibody (1:1000, catalogue number AF564, R&D Systems Inc., US). Blots were probed with antibodies against rabbit anti-rat monoclonal GAPDH (glyceraldehyde 3-phosphate dehydrogenase) antibody (1:10000, catalogue number ab181602, Abcam plc., US) as an internal control. After being incubated with the corresponding horseradish peroxidase (HRP) labelled secondary antibody, the membranes were washed and incubated with SuperSignal West Pico Chemiluminescent Substrate (catalogue number: 34080, Thermo Fisher Scientific Inc., US), and the chemiluminescence signal was detected using the Chemiluminescence Gel Imaging System (Bio-Rad, US).
Statistical analysis
All in vitro experiments of each assay were carried out three times, using the same protocol. Statistical analysis was performed online using the Student's t-test by mean scores with a confidence interval (CI) level of least of 95% on the GraphPad software or the SPSS 13.0 statistical software. A p<0.05 was considered statistically significant.
Results
PRF bioactive membrane promotes rat pressure ulcer healing in vivo
A total of 20 rats were used in this animal model. After three days, the PU area reduced in size in both groups. However, the PU area in the PRF bioactive membrane-treated group was smaller than that of the control group. At day seven, the PU area in the PRF bioactive membrane treatment group was significantly lower (p<0.05) than that of the control model group. After 14 days, the PU area in the PRF bioactive membrane treatment group was almost healed in all rats, and again significantly lower than that of the control group (p<0.05) (Fig 1b, 1c).
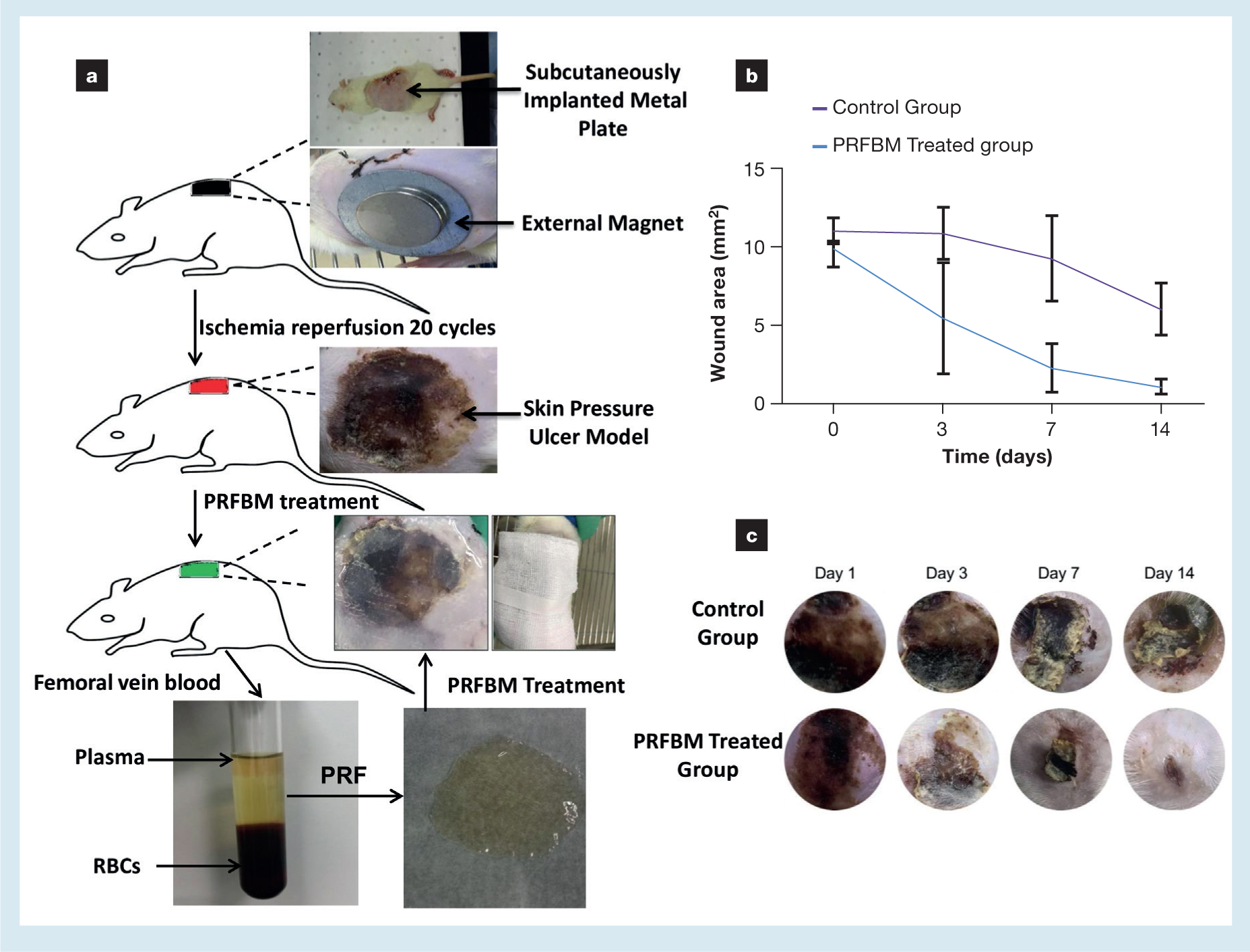
PRF soluble factor extraction promotes RAOEC proliferation in vitro
Viability and proliferation assays were determined in RAOEC (treated with or without PRF soluble factor extraction) at days three, five and seven by using the CCK-8 assay kit. Both the control and PRF bioactive membrane medium could maintain and proliferate viable RAOECs. Different concentrations of PRF soluble factor extraction supplements (5μg, 10μg or 15μg) were added to the culture media, resulting in a significant enhancement of the cell proliferation rate compared with that of the control culture (p<0.05) (Fig 2a).

PRF soluble factor extraction promotes RAOEC migration and wound healing in vitro
RAOECs treated with 5µg, 10µg or 15µg of PRF soluble factor extraction supplement showed significant increases in cell migration compared with the control group (p<0.05; Fig 2b–f). The in vitro wound healing stimuled by the PRF soluble factor extraction was assessed by scratch assays in the RAOEC line. After treatment with or without different concentrations of PRF soluble factor extraction supplements for 24 hours, the scratches that were treated with the 5µg, 10µg and 15µg of total protein from the PRF soluble factor extraction displayed accelerated wound closure compared with untreated control scratches. The cell scratches were almost completely healed after 24 hours of incubation with 15µg of total protein from PRF soluble factor extraction (Fig 3). These data indicate that PRF soluble factor extraction can promote RAOEC's wound healing and migration abilities in vitro.
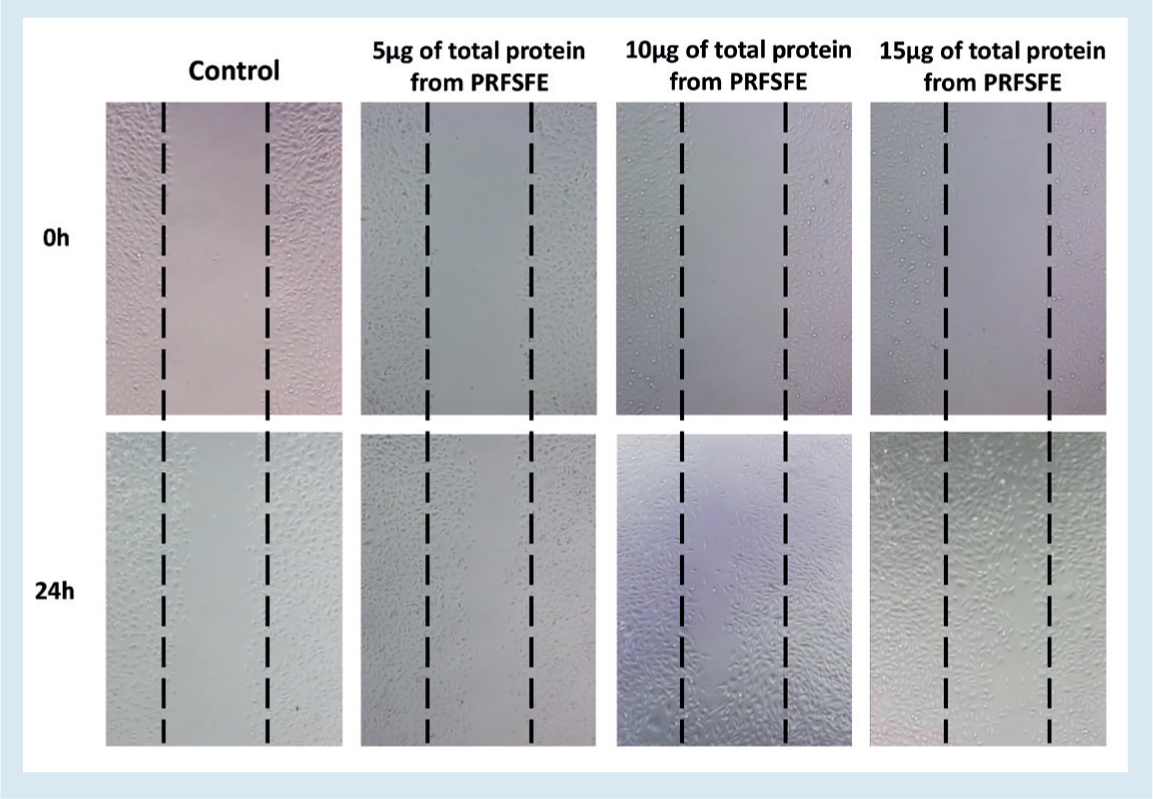
Histopathological changes of rat pressure ulcer models treated with/without PRF bioactive membrane
The histological changes and healing of the two groups' sections were evaluated at days three, seven and 14. There were no differences between the PRF bioactive membrane-treated and control groups at day three post-treatment. Both groups' sections had a stratified squamous epithelium structure that was not clear, lacked skin integrity and showed muscle fibre fracture disorders. However, the sections from days seven and 14 revealed comparable re-epithelialisation between the control and PRF bioactive membrane-treated PUs. The PRF bioactive membrane-treated tissues exhibited a thicker and well-integrated skin, in which the stratified squamous epithelium structure level was gradually healed compared with the control on day seven. On day 14, in the PRF bioactive membrane-treated tissues, the stratified squamous epithelium structure and muscle fibre fracture was completely regrown and healed and had less inflammatory cell infiltration than the control tissue samples (Fig 4).

PRF bioactive membrane promotes rat pressure ulcer healing by increasing VEGF expression
The angiogenic effects of PRF bioactive membrane were evaluated by analysing the VEGF expression levels in PU tissue using the IHC and Western blot (WB) methods. WB results show that after PRF bioactive membrane treatment, the ulcer tissues had a significantly higher expression level of VEGF on days three, seven and 14 compared with the corresponding controls (Fig 5a, 5b). We also performed VEGF IHC staining on rat skin ulcer tissues. On day three, the VEGF expression, based on the IHC results, did not differ between the groups, possibly because the IHC method is less sensitive than the WB method or because the treatment time was insufficient to observe slight changes (Fig 6). However, at days seven and 14 after treatment with PRF bioactive membrane, the IHC data showed a consistent result with WB results, and the treated PU tissue showed a more positive VEGF signal in keratinocytes, which are important in the wound healing process, compared with the control group (as shown by the black arrow, Fig 6).

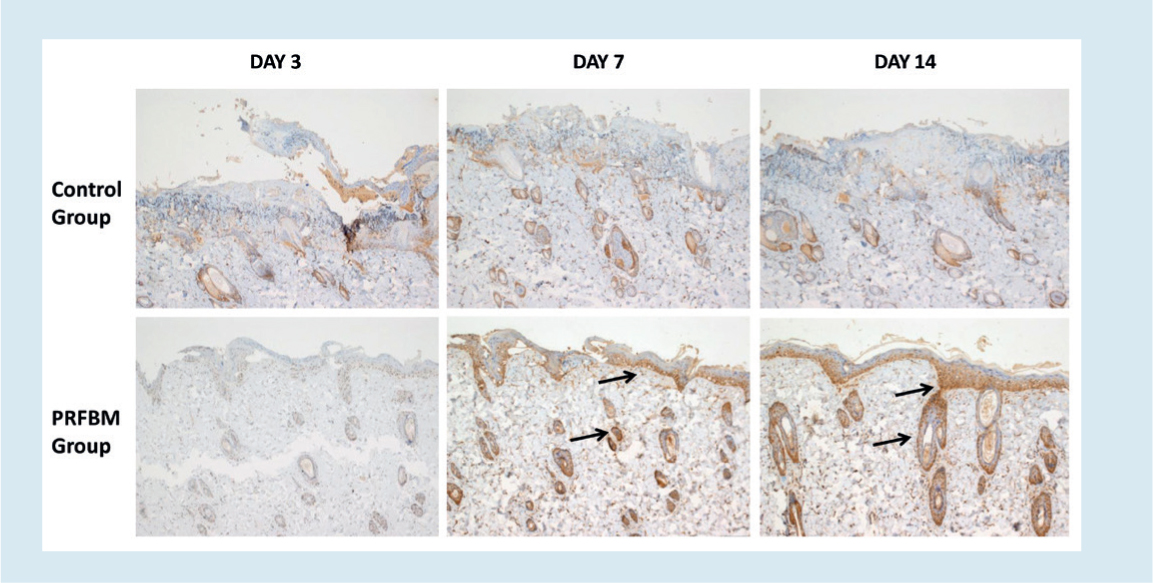
We also tested the VEGF expression changes in rat RAOEC cells that were stimulated with different concentrations of PRF soluble factor extraction supplements. They also showed an increase in VEGF expression in RAOECs after treatment with different concentrations of PRF soluble factor extraction compared with untreated cells; 10μg of total protein from PRF soluble factor extraction had the strongest effects on increasing VEGF expression (Fig 5c, 5d).
Discussion
PUs can be classified into categories I: pink-red area of the skin phase; II: inflammatory infiltration phase; and III and IV: ulceration phase, which is commonly encountered in clinical practice, especially in the ICU. Once PUs occur, they not only increase the patient's distress and impact on their quality of life but can also affect the treatment of any underlying disease, for example diabetes, stroke, hemiplegia, which leads to prolonged hospitalisation and a high risk of infection.7,11 Therefore, it is important to find effective ways to treat PUs.
PRF gel is derived from blood plasma and incorporates leucocytes, platelets and a wide range of healing proteins within a dense fibrin matrix.30 It can serve as a natural bioactive membrane (PRF bioactive membrane), which can enhance soft and hard tissue healing. At the same time, it can also protect surgical sites and grafted materials from external aggressions, and the growth factors released from PRF bioactive membrane can activate a cascade of signal-pathways related to tissue repair.30,31 It has been applied to wounds to promote tissue regeneration and repair in different surgical fields, such as plastic surgery,28,32,33 orthopaedics34 and dentistry.21,23,24,28,35 Furthermore, Greer et al.36 evaluated the effectiveness of using PRF to treat patients with chronic illnesses such as diabetes. Despite the promising preclinical findings and the huge interest in the clinical application of PRF, it has few applications for the treatment of PUs and its underlying mechanism is still unknown. In this animal model, a PU was created and treated with a fresh application of PRF bioactive membrane every three days. After 14 days of treatment, the PUs treated with PRF bioactive membrane had healed. Different concentrations of PRF soluble factor extraction could significantly promote migration and wound healing of RAOEC cells in vitro. These data indicate that PRF bioactive membrane can promote PU healing, partly due to its ability to promote endothelial cell proliferation and migration.
Growth factors are powerful molecules, and small variations in their concentration can have a significant effect on angiogenesis, which is essential for healthy tissues. Previous studies demonstrated that growth factors play an important role in cell proliferation, differentiation, tissue repair, ulcer formation, and wound healing.37,38–39 VEGF is recognised as a key factor in regulating angiogenesis in the ulcer healing process.40 Accumulating evidence has established the fundamental role of VEGF as an important regulator of normal and abnormal angiogenesis over the last decade.37 It is a chemotactic agent and a potent endothelial cell mitogen that also influences vascular permeability. It is expressed in organs during embryogenesis and, to a limited extent, in adult organs, such as the circumventricular organs.41 However, in disease states, VEGF is detected in various tumour cells and keratinocytes during the wound healing process.41,42 Based on the promoting effects of PRF bioactive membrane and PRF soluble factor extraction on PU healing and endothelial cell proliferation and migration both in the in vivo and in vitro experiments in this study, changes in VEGF expression were further tested both in vivo and in vitro. After stimulation with different concentrations of PRF soluble factor extraction in RAOEC cells, VEGF expression was markedly increased. In agreement with the in vitro results, compared with the control group, the VEGF expression level was increased in PRF bioactive membrane-treated rat PU tissues. These results suggest that PRF bioactive membrane promotes PU healing, possibly due to the cascade reaction of VEGF induction.
Limitations
The animal model demonstrated that PRF bioactive membrane could promote healing in rat PUs, the study has limitations. Due to the complexity of the clinical causes of PUs, this artificial model cannot fully simulate the PUs caused by various reasons. Therefore, it may not be possible to demonstrate a good therapeutic effect in all patients with PUs using this method. Further studies on the application of this method and its efficacy in the treatment of PUs in humans is required.
Conclusion
PRF bioactive membrane has been shown to significantly accelerate the healing of rat PUs and may be a new tool for treating PUs in humans. It is possible that the bioactive membrane could be produced by a standardised procedure, using components derived from the patient, ensuring biocompatibility, complete biodegradation and resorption of the PU in clinical practice.